The challenges of antiviral treatments
Physician Claudette Poole doesn't take long to rattle off a list of antiviral medications she prescribes to her patients. "There really aren't very many," says Poole, a pediatric infectious disease physician at the University of Alabama at Birmingham.
And for people with Covid-19, there's just one approved for use: remdesivir, which doesn't seem to save lives, but speeds recovery in those who do get well. Clearly, more antivirals would be nice to have — so why don't we have them? Inventing them, it turns out, is not so easy.
Viruses rely on human cell machinery to copy themselves, so antiviral designers face a challenge: how to stop the virus without damaging the inner workings of healthy cells too. While scientists have found several solutions to the problem, the antiviral pharmacopeia still lags behind the plethora of antibiotics available to treat bacterial infections.
But as researchers build up their knowledge of viral life cycles, antivirals may be poised to catch up. Scientists are also planning for future pandemics, in the hopes of having a better selection of antivirals to try the next time around.
Here's where antivirals stand today, and how the list might be growing.
How do antivirals work?
An antiviral drug can block any of the steps a virus uses to copy itself. To do its dirty work, a virus must attach to a host cell, sneak inside and trick that cell into copying viral genes and crafting viral proteins; after that, the newly made viruses must escape to infect new targets. At each step, viral genes or proteins need to interact with various host molecules, and each of these interactions offers an opportunity for antiviral drugs. The drugs often mimic those host molecules and act as decoys to interfere with the viral life cycle and reduce its spread.
A common approach is to interfere with the copying of viral genes into DNA or RNA to form new viral genomes. Viruses frequently have their own versions of proteins, called polymerases, for this task. The polymerases add individual building blocks called nucleotides, one by one, to the new genome as it's being built.
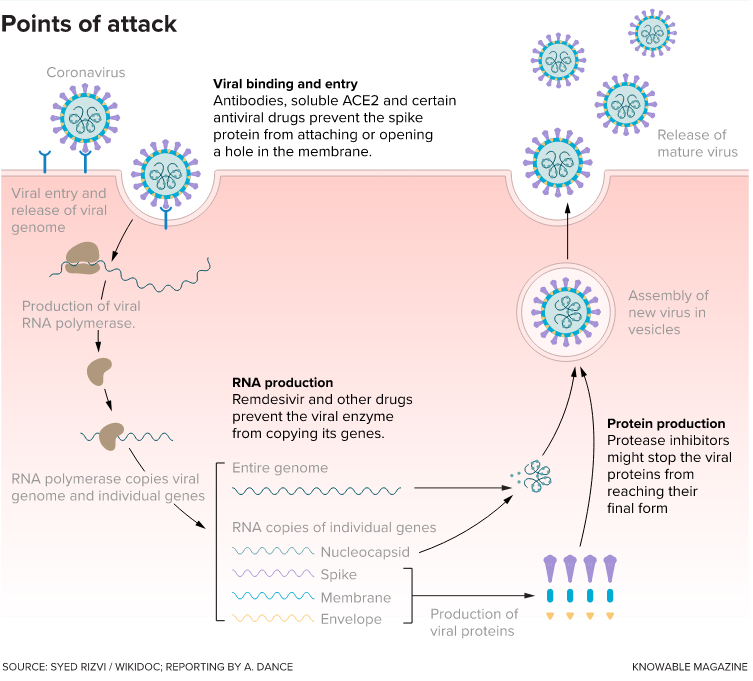
Drugs that block viral binding and entry make the virus less able to invade host cells. Other drugs (including remdesivir, the only
antiviral currently approved in the US for treating Covid-19) prevent the virus from making new copies of viral RNA. A third set of drugs
interferes with production of the proteins required to form complete, functioning new viruses.
For example, the drug acyclovir, used to treat herpes, goes after this genome-copying step. To the virus's polymerase, the medicine looks like just another building block — but it's not. Once the decoy gets into the growing strand, it prevents the addition of any more nucleotides. For the virus, it's game over.
Another drug, oseltamivir (Tamiflu) for influenza, acts at the stage of viral exit from the infected cell. The virus uses a key protein called neuraminidase to dissolve its way out, but oseltamivir sticks to the neuraminidase and stops it from working.
Since antivirals don't eradicate viruses directly — they just stop them from spreading cell to cell or person to person — it's up to the body's immune system, when possible, to mop up the invaders already present. That's why it's important to start antiviral treatment early, while viral numbers remain low. "The faster you can take the drug, the more you can limit the virus's ability to spread," says virologist Mark Heise of the University of North Carolina at Chapel Hill. Tamiflu, for example, works best when taken within 48 hours of the first symptoms, helping people recover about a day faster.
Why are there so few antivirals?
The number of antivirals is paltry compared with the list of bacteria-fighting antibiotics. That's due to several factors.
For one, antibiotics were first out of the starting gate, notes Erik De Clercq, an emeritus professor of biomedicine at KU Leuven in Belgium. The first, penicillin, was discovered in 1928 and first used in a patient in 1940. In contrast, the first antiviral, idoxuridine, was developed as an anticancer agent in 1959, was reported to block viruses in 1961, and approved in 1963 to treat herpes infections of the eye. (De Clercq, a leader in early antiviral research, described his scientific journey in the Annual Review of Pharmacology and Toxicology in 2011.)
Plus, viruses are much trickier targets than bacteria, says Monica Gandhi, an infectious disease physician at the University of California, San Francisco. Bacteria are whole living cells with all the metabolic pathways they need for survival, so they offer plenty of targets for attack. They also have unique features such as cell walls that aren't found in human cells. That means antibiotics can interfere with cell walls, or other bacteria-specific parts and processes, to kill the pathogens without harming our own cells. And because microbes evolved antibiotics to battle each other, there is a diverse array of the compounds out in nature.
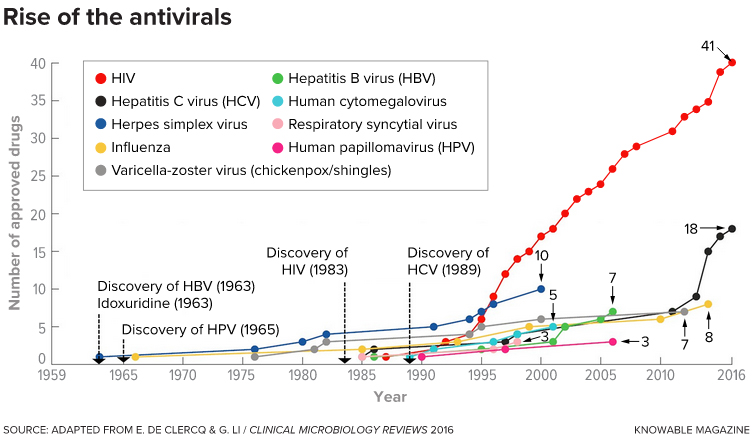
as this timeline shows. Drugs to treat HIV infections are the clear exception, though drugs against hepatitis C virus also have begun to flourish.
The timeline also shows the date of discovery of four of the nine viruses; the other five were discovered before 1959.
(Drug numbers are as of 2016.)
In contrast, viral pathogens live inside our own cells and depend on our proteins for most of their needs, so they offer no such easy targets. And few natural antivirals exist, so scientists need to invent them from scratch, says Kathie Seley-Radtke, a medicinal chemist at the University of Maryland, Baltimore County.
Moreover, antivirals have a limited number of possible shapes. That's because, to block a virus's actions, they must fit into viral proteins as decoys.
The biggest challenge, says Seley-Radtke, is to ensure that the drugs don't hurt the human hosts as well. For example, in the case of nucleotide mimics like acyclovir, wouldn't there be a risk that they would get into the cell's DNA as well as the virus's?
There are ways around that problem. In the case of acyclovir, the drug that patients swallow is an inactive form, and it is mainly activated by a viral protein. "It targets the virus very nicely, while leaving host cell DNA alone," says Poole, who reviewed the use of acyclovir and other antivirals for newborns with herpes simplex virus in the 2018 Annual Review of Virology.
There is one unfortunate similarity between antibiotics and antivirals: In both cases, pathogens can make tiny changes to their genes and proteins that leave them unharmed by the drug. "Resistance is a huge issue in antivirals," says Poole. Doctors used to prescribe drugs called adamantanes to people with the flu, for example — but the influenza viruses circulating among people today are unaffected by the drugs. "They are no longer effective at all," says Poole. "There's a desperate need to find other flu antivirals."
The one exception to the dearth of antivirals is the flourishing pharmacopeia of meds against the human immunodeficiency virus, resulting from decades of research. Gandhi says that she can select from 30 or so medications to treat her HIV-positive patients. More are on the way — and just as well, because HIV can quickly evolve resistance to any one drug.
"HIV research set the tone," says Heise, and it's bringing other antivirals in its wake. "We're seeing, again, new antivirals coming out much more quickly over the last several years." In the last five years, for example, novel medications have turned hepatitis C from a chronic to curable condition.
"I think we'll see more drugs for acute viral infections coming forward as well," says Heise.
How are antivirals being used against Covid-19?
Drugs designed against one virus often work against others, because proteins such as the polymerases used to copy virus genomes are similar across a wide range of viruses. But the current scourge requires more than the usual amount of cleverness from antiviral designers.
"Coronaviruses are pretty tricky," Seley-Radtke says. Simple nucleotide mimics like acyclovir won't work, because these viruses have another protein that acts as an editor, monitoring the polymerase's work, recognizing the decoy and cutting it out.
Enter remdesivir, which had already been tested in people with Ebola (though it didn't help them much). It's a nucleotide mimic, but it's a bit special. Once incorporated into a piece of the new viral genome — which in the case of coronaviruses is made of RNA — it doesn't stop the strand's growth right away. The polymerase keeps adding normal nucleotides. But after it's added a few, the drug bends the RNA strand so badly that the polymerase can't keep building. By then, though, the coronavirus editor protein no longer works; the normal nucleotides added after remdesivir seem to get in the way, says Seley-Radtke. Thus, the polymerase is stuck.
Despite that clever trick, remdesivir's performance in people with Covid-19 has been decidedly lukewarm. On the plus side: In a trial of 1,062 people hospitalized with the virus, those who were treated with remdesivir recovered more quickly than those who received an inactive placebo. Based on this and two similar studies, the US Food and Drug Administration authorized remdesivir to treat hospitalized patients.
But what remdesivir doesn't seem to do is save lives. In November, the World Health Organization, citing its own larger but preliminary study, recommended against remdesivir for hospitalized patients for the time being, until more research can be done. The WHO noted "no evidence that remdesivir improves survival and other outcomes."
The results, which at first blush may seem confusing, make sense considering how antivirals work, says Poole. Because remdesivir requires multiple intravenous infusions, it's given only to hospitalized patients. But by the time a person with Covid-19 is ill enough to be hospitalized, the virus has already run rampant across their body, so remdesivir comes too late to do much good. "The game-changer," she says, "is going to be when we find an antiviral that you can give to people orally before they get into the hospital."
Remdesivir's maker, Gilead Sciences, is working on an inhaled version. And there are other antivirals in the pipeline. For example, Seley-Radtke is optimistic about another nucleotide analog, known as AT527. Under joint development by Roche and Atea, AT527 is now midway through human trials. Like remdesivir, it has delayed action, so it avoids being edited out of a growing RNA strand. But unlike remdesivir, it's a pill you can swallow. The companies hope it can be used by hospitalized and non-hospitalized patients alike, and perhaps even be taken by people exposed to Covid-19 to prevent infection from taking hold to begin with.
The pandemic has sent scientists scrambling to find treatments. Heise, for one, is testing a wide range of drugs — not just standard antivirals — against SARS-CoV-2 in lab dishes, as part of the Rapidly Emerging Antiviral Drug Discovery Initiative (READDI). The idea is that, because the virus depends on many processes in human cells, a variety of medications that act on human proteins might give doctors an edge by hurting the virus more than the patient. That throws the doors open to considering medications that were originally designed for cancer, psychosis, inflammatory conditions and autoimmune disease, to see if they might have a shot against Covid-19.
But the READDI collaborators — including academic centers, pharmaceutical companies and nongovernmental organizations — are aiming for more than a Covid-19 treatment. READDI hopes to identify and test potential medications for as-yet-unknown infections that may crop up in the future.
By getting early human safety testing done ahead of time, they'll be ready to spring into action when those future outbreaks happen. As Heise says, "We don't want to repeat what we've just been through."
Amen to that.
This article is part of Reset: The Science of Crisis & Recovery, an ongoing series exploring how the world is navigating the coronavirus pandemic, its consequences and the way forward. Reset is supported by a grant from the Alfred P. Sloan Foundation.
Enjoy reading ASBMB Today?
Become a member to receive the print edition four times a year and the digital edition weekly.
Learn moreGet the latest from ASBMB Today
Enter your email address, and we’ll send you a weekly email with recent articles, interviews and more.
Latest in Opinions
Opinions highlights or most popular articles
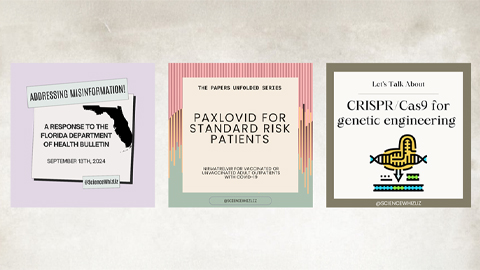
Leveraging social media to share science
Scientist and educator Elisabeth Marnik explains how to combat misinformation, such as the popular myth that drinking bleach will prevent infections.
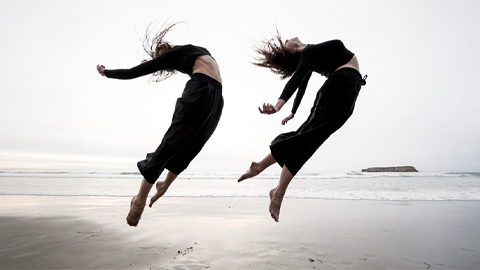
Dancing cancer
A molecular biologist and a choreographer describe how they came to work together.
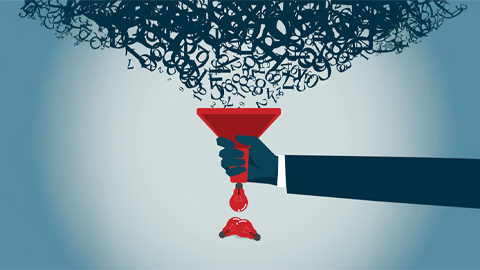
Can AI help people trust scientists?
Scientists use jargon and complicated language to describe their work. Regular folks ‘get it’ more when descriptions are simpler – and think better of the researchers themselves.
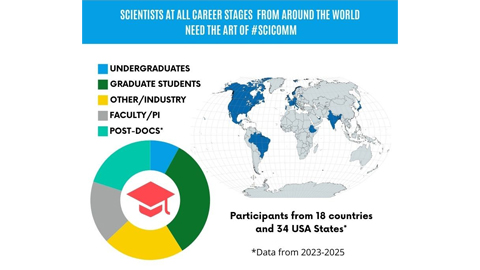
The Art of Science Communication as an infographic
Sometimes a picture is worth a lot of words.
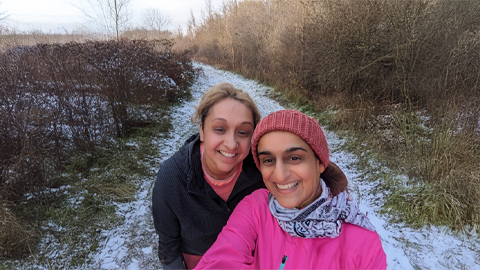
Guiding my sister through cancer
A scientist learns that sometimes communicating all the data and research needs to take a backseat.
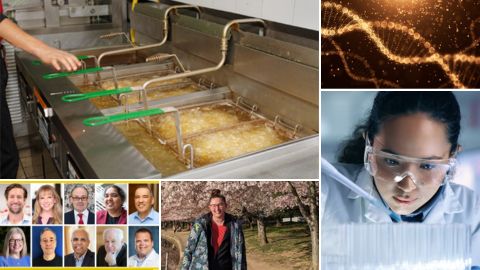
Our top 10 articles of 2024
ASBMB Today posted more than 400 original articles this year. The ones that were most read covered research, society news, policy, mental health, careers and more.