Why scientists are studying
if chloroquine
could treat coronavirus
As COVID-19 ravages the world, scientists are desperately trying to develop a medication to stop the virus (severe acute respiratory syndrome coronavirus 2, or SARS-CoV-2). Dozens of drugs and vaccine candidates are in various stages of development and testing. Among these is chloroquine, a seemingly strange choice as it has been widely used to treat malaria since the 1940s.
Editor's note: Do not take any form of chloroquine or hydroxychloroquine without medical supervision.
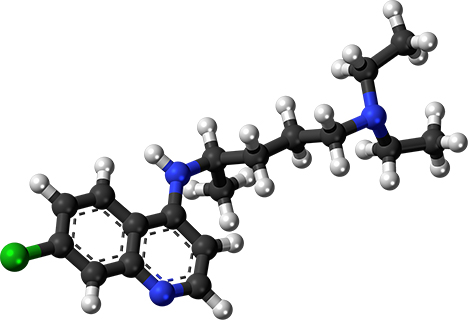
Not only is chloroquine effective in treating malaria, it is inexpensive to make and remarkably well-tolerated by most patients (though it poses the same dangers all medications do if taken without the oversight of a doctor). It was such a good drug in the battle against malaria that it was overused, facilitating the emergence of malaria parasites that are resistant to it.
Malaria is caused by a single-celled parasite called Plasmodium. After a mosquito harboring the parasites bites a victim, the parasites make their way to the liver and eventually the red blood cells. Inside red blood cells, the parasites use the hemoglobin as a food source, which is digested in the parasite’s acidic food vacuole. The eventual destruction of red blood cells leads to life-threatening anemia. Consequently, malaria claims the lives of over 400,000 people per year, mostly children under the age of five in sub-Saharan Africa.
How chloroquine kills Plasmodium parasites has long been the subject of research. The drug is capable of binding to heme, which is a toxic byproduct of hemoglobin digestion. The parasite avoids the toxic effects of heme by stacking the molecules into an inert crystal called hemozoin. But when chloroquine binds to heme, hemozoin cannot be made, forcing the parasite to die in its own waste. Further supporting this idea is the fact that chloroquine concentrates in acidic cellular compartments, such as the parasite’s food vacuole.
But a virus is nothing like Plasmodium, begging the question of how chloroquine could possibly affect it. A virus is not even a cell — it is merely a small strand of DNA or RNA encapsulated in a bubble-like membrane of lipid and protein. After viruses invade, they co-opt the host cell’s machinery to reproduce themselves. They are the ultimate unwanted guest, depleting the host cell’s resources to make copies of themselves — virus babies, if you will.
The exploration of chloroquine as a potential antiviral agent has been ongoing for quite some time. A study in 2005 showed that chloroquine prevented the spread of SARS CoV in cultured cells (SARS CoV is a similar respiratory virus that caused the 2002 outbreak).
A study this February showed that chloroquine inhibited SARS-CoV-2 in cultured cells as well as a small cohort of about 100 patients with COVID-19. In addition, a new clinical trial underway in France recently released news that a combination drug therapy employing chloroquine showed significant reduction of the virus in the 20 patients treated so far.
So how does chloroquine thwart coronavirus? When a virus replicates inside a host cell, its pieces get made and processed by the host cell’s manufacturing system. A major part of this protein processing center is a compartment called the Golgi apparatus, which releases those proteins within acidic vesicles called endosomes. Endosomes then deliver this protein cargo to other places, including the cell surface. Viruses can hijack this system to process and release their babies from the host cell. In addition, SARS CoV enters a host cell through an endosome vesicle formed from the host cell’s surface membrane.
Recall that chloroquine concentrates inside acidic cellular compartments. It can get into Plasmodium’s food vacuole, but it also concentrates inside a cell’s endosomes, which are used by viruses both when they enter and exit a host cell. Chemically, chloroquine is a weak base, sufficient to raise the pH in these compartments. As endosomes become less acidic, the proteins inside are ruined — they unfold because they are no longer at the correct pH. What this means for coronavirus is that its viral proteins are rendered nonfunctional because chloroquine changed the pH of the endosomes carrying them.
In addition to ruining the viral invader’s proteins, chloroquine can distort the shape of proteins called cytokines, which can reside in the endosomes of immune cells that fight infection. Sometimes, the immune system gets too excited and creates what is called a “cytokine storm,” which has been a major complication of COVID-19. By quelling this cytokine storm, chloroquine provides a dual advantage in helping the body combat coronavirus infection.
Chloroquine has been used for decades and has a reputation for being extraordinarily safe, although it is not used in patients with epilepsy, myasthenia gravis, or glucose-6-phosphate dehydrogenase deficiency. Chloroquine can also cause severe reactions in patients with psoriasis.
This would not be the first time chloroquine has been “repurposed” to treat a condition other than malaria. As noted, chloroquine can settle the immune system; in other words, it has anti-inflammatory properties. Consequently, the drug has been useful in treating inflammatory diseases, such as rheumatoid arthritis and lupus erythematosus.
If these promising initial results continue to hold up, chloroquine could possibly offer a cheap and safe treatment for those suffering from COVID-19.
Enjoy reading ASBMB Today?
Become a member to receive the print edition four times a year and the digital edition weekly.
Learn moreGet the latest from ASBMB Today
Enter your email address, and we’ll send you a weekly email with recent articles, interviews and more.
Latest in Science
Science highlights or most popular articles
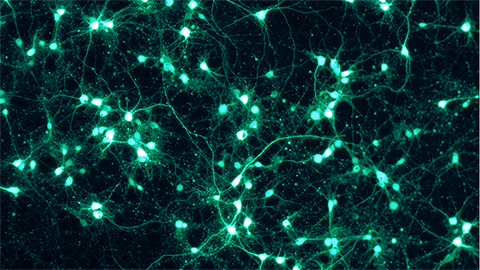
Adults grow new brain cells
How does the rare birth of these new neurons contribute to cognitive function?
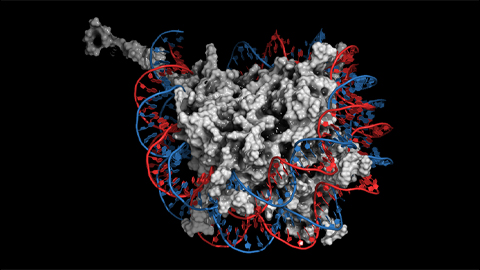
From the journals: JBC
Histone demethylase inhibited by own sequence. MicroRNA reduces cell cycle–related apoptosis. Multipurpose antibiotic takes on staph infections. Read about recent JBC papers on these topics.
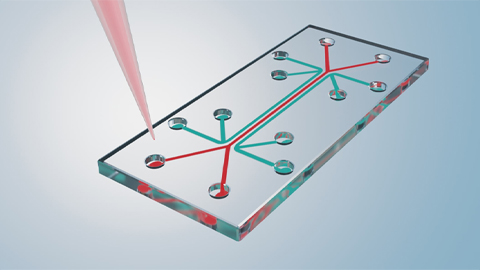
Tiny laboratories that fit in your hand can rapidly identify pathogens using electricity
Pathogens have distinct electrical charges, shapes and sizes. Measuring how quickly they move through an electric field can help researchers separate different species in a sample.

Toxoplasma gondii parasite uses unconventional method to make proteins for evasion of drug treatment
This recent study by a team from Bill Sullivan’s lab at the Indiana University School of Medicine was named a Journal of Biological Chemistry Editor’s Pick.
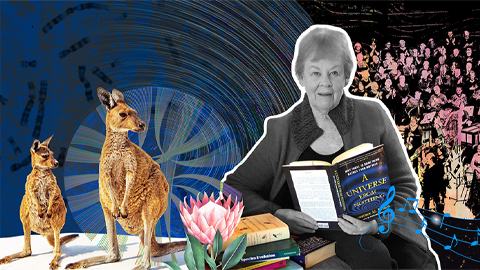
Of genes, chromosomes and oratorios
Jenny Graves has spent her life mapping genes and comparing genomes. Now she’s created a musical opus about evolution of life on this planet — bringing the same drive and experimentalism she brought to the study of marsupial chromosomes.
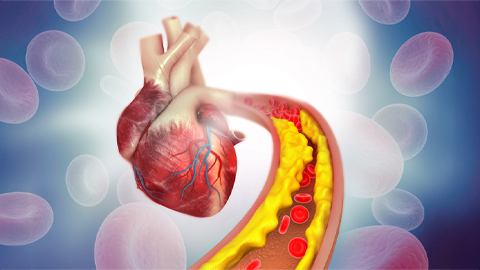
Ubiquitination by TRIM13: An ingredient contributing to diet-induced atherosclerosis
Researchers help unravel the molecular mechanism behind plaque formation in cardiovascular disease.