Tapping into bacterial conversations
Over 500 years ago, scientists discovered bacteria: single-celled organisms that likely first appeared on Earth some 3.5 billion years ago. But, it took until the 1970s for researchers to begin to uncover how these seemingly primitive lifeforms carry out sophisticated, coordinated behaviors. This discovery revealed that bacteria work together – for both good and for bad – and it also paved the way for applications that might one day be used to combat antibiotic resistant pathogens of global importance.
Through a process now known as quorum sensing, bacteria communicate with one another using a chemical “language.” For more than three decades, HHMI Investigator Bonnie Bassler has dedicated her life’s work to decrypting this language.
Bacteria reproduce through a process in which a single bacterial cell essentially doubles in size before splitting into two daughter cells. As they grow, bacteria also make and release small molecules called autoinducers, and the amount of autoinducer molecules in the vicinity increases in step with the increasing number of bacterial cells.
Once the amount of the autoinducer molecules hits a certain threshold, the bacteria detect their presence and infer from the buildup of autoinducers that other bacteria must be nearby. In this way, bacteria use autoinducers to take a census of the population. Further, autoinducer detection cues the bacteria to simultaneously change their behaviors; they stop acting as individuals and instead work together to carry out tasks that take many bacteria working in synchrony to make the tasks successful.
“My lab tries to figure out how bacteria get any bang for their buck,” Bassler says. “They are puny, yet they have the power to make us sick, and increasingly we understand that bacteria also have the capability to be beneficial as every higher organism has bacteria that live in and on them, and they help keep us alive.”
Bassler says the question her lab asks isn’t whether bacteria can accomplish powerful tasks, but rather, how can they manage to do so given that bacteria are so small, and individually, inconsequential.
“We’ve shown that quorum sensing gives bacteria profound importance in nature,” she says. “Bacteria communicate with chemical words, they count their numbers, they act in groups, and they carry out tasks as collectives that they could never accomplish as individuals because, individually, each bacterium is too small to make a difference,” she says.
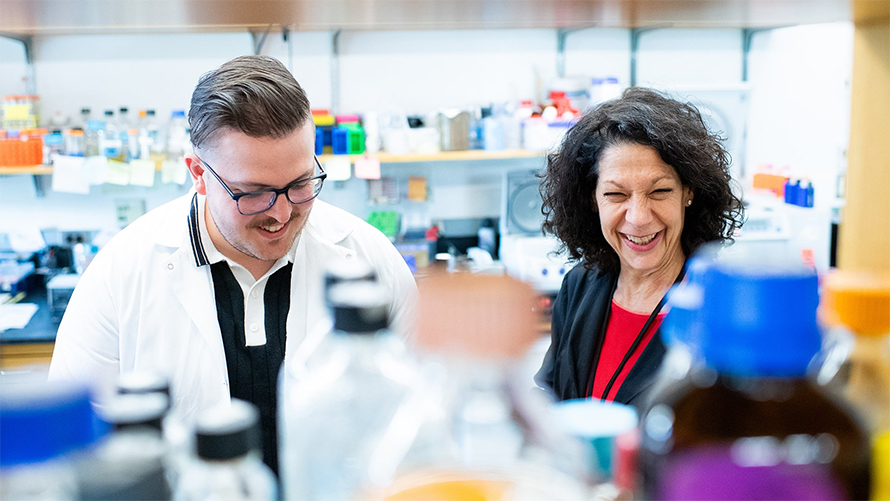
Step foot into Bassler’s lab at Princeton University and you’ll quickly see how this discovery shapes every aspect of her group’s work, far beyond the realm of bacteria.
As an undergraduate student at UC Davis, Bassler once scoffed at the idea of studying bacteria. But she stumbled into her first opportunity to work in a lab thanks to a bulletin board with a flyer from Frederic A. Troy II, a professor in the medical school.
At the time, Bassler had no research experience; she had enrolled in college on a mission to become a veterinarian, only to find it wasn’t the right fit. Fortunately, the program’s course requirements opened Bassler’s eyes to the worlds of biochemistry, genetics, and molecular biology. She was enthralled with the subjects and jumped at the opportunity when she saw that Troy’s lab needed undergraduates to support one of two projects: a cancer research project, and a bacterial research project.
“I was 19 years old, and I thought, ‘Oh, I’m going to cure cancer if I go join his lab.’ I was so ridiculous. But he put me on the bacterial project – and I was absolutely appalled!” Bassler recalls, laughing. “Then I thought, ‘Oh, this is a test. If I show I’m earnest and hard-working, eventually he’ll transfer me to the cancer project.’ Well, now it’s a ‘few’ years later, and I’m still working on bacteria.”
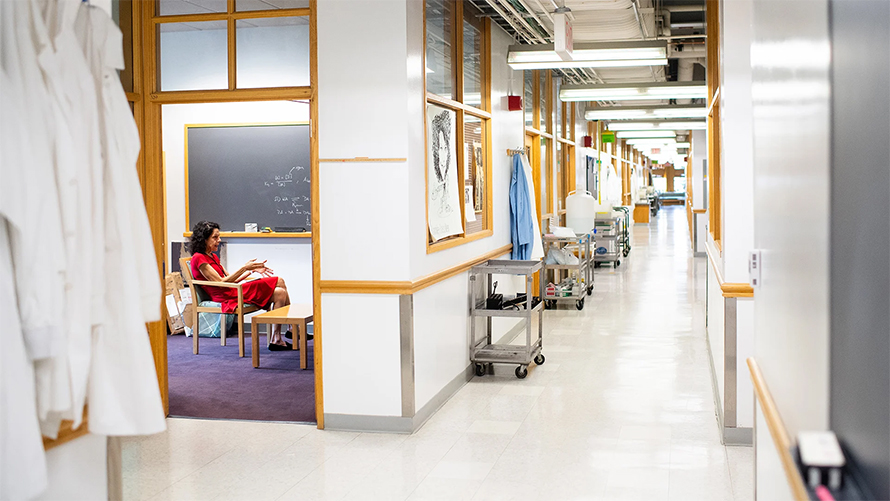
Elbow-to-elbow
In the 1970s, Woody Hastings, a biochemist at Harvard University, laid the groundwork for the field of quorum sensing research by studying an obscure bioluminescent marine bacterium called Vibrio fischeri. The bacterium had the curious behavior of only turning on light production at high cell density. Together with his student, Kenneth Nealson, he described the phenomenon of an autoinducer molecule building up and signaling to the bacteria to collectively produce bioluminescence.
In the mid-1980s, Mike Silverman, principal scientist at the Agouron Institute, entered the scene with graduate student JoAnne Engebrecht. Together, they identified the genes involved in the Vibrio fischeri signaling pathway that controlled collective bioluminescence, and they characterized their functions, establishing the model that still exists today for how quorum sensing works.
“Mike Silverman broke things open,” Bassler says.
In 1989, Bassler learned of Silverman’s work when she attended her first-ever science meeting. There, Silverman gave a talk on Vibrio fischeri and the notion of bacteria being able to act as a group. Bassler, an intimidated 20-something-year-old, marched up to the podium afterwards. “I said to him, ‘You just have to let me be your postdoc.’”
Eventually, he said yes.
Bassler got to the lab in 1990 and found only one other person working on quorum sensing, and shortly after Bassler’s arrival, that other lab member left.
“I was like, ‘Where is everybody?’ and Mike said, ‘You are everybody!’” she laughs. “It was amazing, because I got to work elbow-to-elbow with him.”
The experience – and the partnership – forever changed the course of Bassler’s life.
“He always believed in me,” she says. “He would always tell me, ‘Oh, you’re just around the corner. I can tell you’re pretty close.’ Now that I’m in his position, as leader of the lab, I realize he was just saying that to keep my spirits up because the projects break open in their own time. He was really positive, both about the fun of doing science, and the thrill of discovery. And, he taught me to be a really rigorous scientist.”
Silverman’s encouragement and optimism stayed with Bassler, and helped shape her own approach to mentorship.
“He taught me that the science is going to be challenging if you’re doing it right,” she says. “It ought to be difficult or somebody else would have already made the discovery. He helped me realize it can be so fun and so challenging and so meaningful all at the same time.”
At Princeton, Bassler and her team showed that quorum sensing is the norm in the bacterial world: the process is not restricted to bioluminescent bacteria. In fact, bacteria use multiple autoinducers to communicate, and these molecules harbor information not only about how many bacteria are in the community, but also about how closely or distantly neighbors are related to one another.
Building on this, Bassler and her team showed that bacteria can distinguish “self” from “other” using quorum sensing, and they accordingly tailor their behaviors based on whether they are surrounded by friend or foe.
Bassler’s group also found that higher organisms – including human cells and viruses – participate in quorum sensing conversations. They discovered that disrupting quorum sensing communication in pathogenic bacteria renders these bacteria incapable of infection, and this finding has paved the way for new biomedical applications.
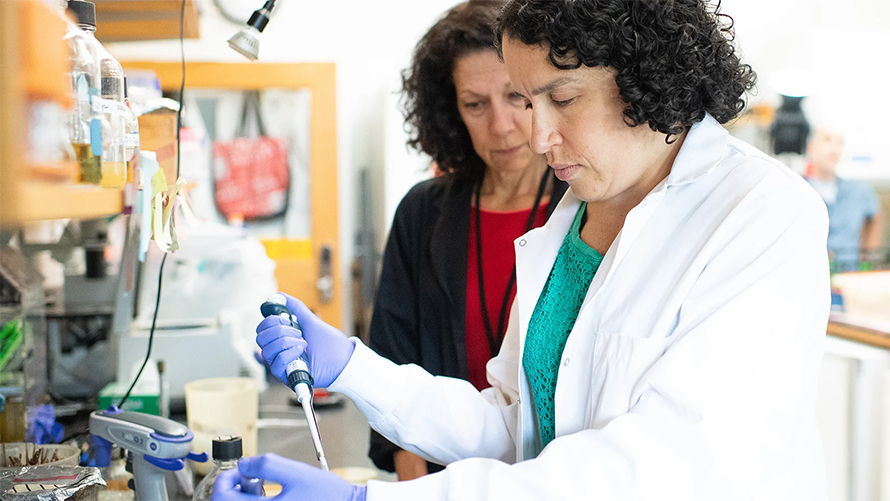
Working in tandem
“There’s so much science happening in the US and around the world,” says Frank Santoriello, a postdoctoral researcher in Bassler’s lab. “What you need to realize when you’re coming up is that there’s good science everywhere. There’s not this one lone genius who’s going to change your world. In a quorum sensing lab especially, we realize that working together is a lot better than working as an individual. Working here with Bonnie and the people she’s surrounded herself with is the best thing about being here.”
“Even though many of us are working on different projects, we all have a similar goal in mind: to better understand quorum sensing and how bacteria communicate,” adds Grace Beggs, a postdoctoral researcher in Bassler’s lab.
Bassler and her team speak humbly, but in many ways, their work – and that of others before them – has generated an entirely new and unexpected branch of science that centers on how collective behaviors evolved on Earth. Their work is the result of questions raised years ago about the basics of bacteria, but it has the potential to lead to applications that will change aspects of everyday life.
“I think research in cell-cell communication is exciting because it can drive so many future discoveries on how these bacteria work, what’s helping them to determine how they should behave, and, importantly, how we need to go about treating them if they’re causing disease,” says Julie Valastyan, a research associate in Bassler’s lab.
In the world of medicine, quorum sensing has piqued the attention of many. If scientists could develop strategies to block the signaling between a cell’s receptor and a particular autoinducer molecule, they could potentially prevent harmful bacteria from working together to cause infection. In this way, doctors might one day be able to administer anti-quorum sensing therapies that shut down or prevent infections without wiping out beneficial bacteria in the process.
Still, the field is young. And, although quorum sensing offers promise for alternatives to traditional antibiotics, such revolutions in medicine are a long way away, Bassler says.
But, the fact that many labs are already focused on making such therapies possible speaks volumes about the work carried out by Bassler and her lab, and those who first inspired them.
“I thought I’d figure all this out during my career,” Bassler says, reflecting on her decades of discoveries in quorum sensing. “I thought I would solve this, and I’m not even close. And so on the one hand, that’s sort of, sad for me, right? Because I won’t be around long enough to figure out all I want to know about quorum sensing. But on the other hand, that’s so exciting because it means we’re working on something that’s worthy of our time and effort. It means that tapping into quorum sensing is a huge challenge that has important ramifications that we never anticipated early on, or even in the middle of my career. It means the field is flourishing, that it’s expanding instead of contracting.”
With a smile, Bassler adds, “That’s the greatest kind of career one could have – where you’re still running as fast and as hard as you can, and not getting to the finish line.”
This article is republished from HHMI News & Stories. Read the original here.Enjoy reading ASBMB Today?
Become a member to receive the print edition four times a year and the digital edition monthly.
Learn moreGet the latest from ASBMB Today
Enter your email address, and we’ll send you a weekly email with recent articles, interviews and more.
Latest in Science
Science highlights or most popular articles
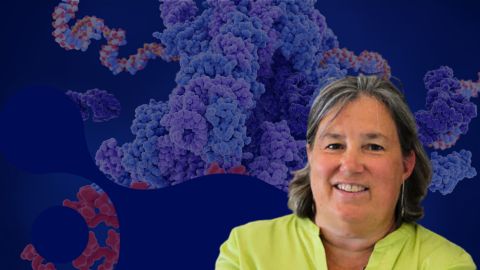
Melissa Moore to speak at ASBMB 2025
Richard Silverman and Melissa Moore are the featured speakers at the ASBMB annual meeting to be held April 12-15 in Chicago.
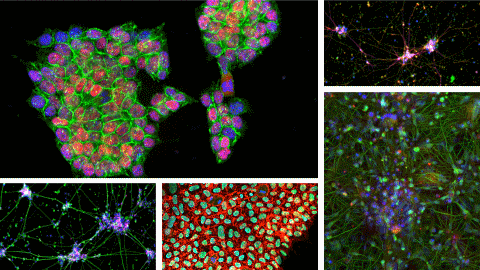
A new kind of stem cell is revolutionizing regenerative medicine
Induced pluripotent stem cells are paving the way for personalized treatments to diabetes, vision loss and more. However, scientists still face hurdles such as strict regulations, scalability, cell longevity and immune rejection.
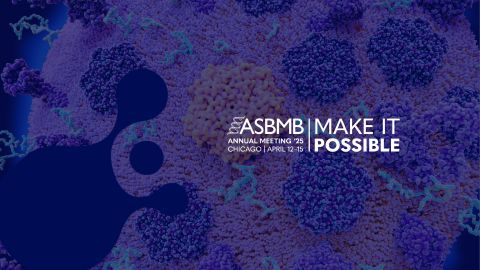
Engineering the future with synthetic biology
Learn about the ASBMB 2025 symposium on synthetic biology, featuring applications to better human and environmental health.
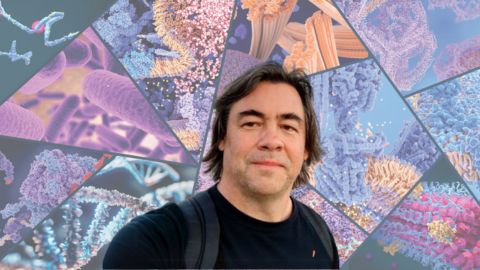
Scientists find bacterial ‘Achilles’ heel’ to combat antibiotic resistance
Alejandro Vila, an ASBMB Breakthroughs speaker, discussed his work on metallo-β-lactamase enzymes and their dependence on zinc.
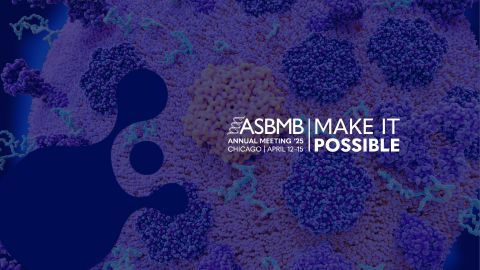
Host vs. pathogen and the molecular arms race
Learn about the ASBMB 2025 symposium on host–pathogen interactions, to be held Sunday, April 13 at 1:50 p.m.
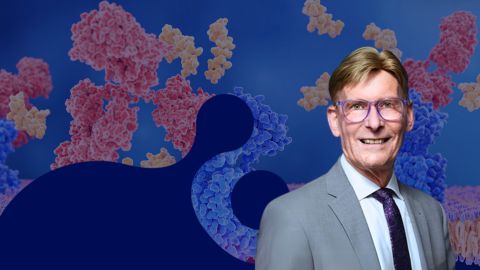
Richard Silverman to speak at ASBMB 2025
Richard Silverman and Melissa Moore are the featured speakers at the ASBMB annual meeting to be held April 12-15 in Chicago.